This article has been reviewed according to Science X's editorial process and policies. Editors have highlighted the following attributes while ensuring the content's credibility:
fact-checked
peer-reviewed publication
trusted source
proofread
Building an understanding of quantum turbulence from the ground up
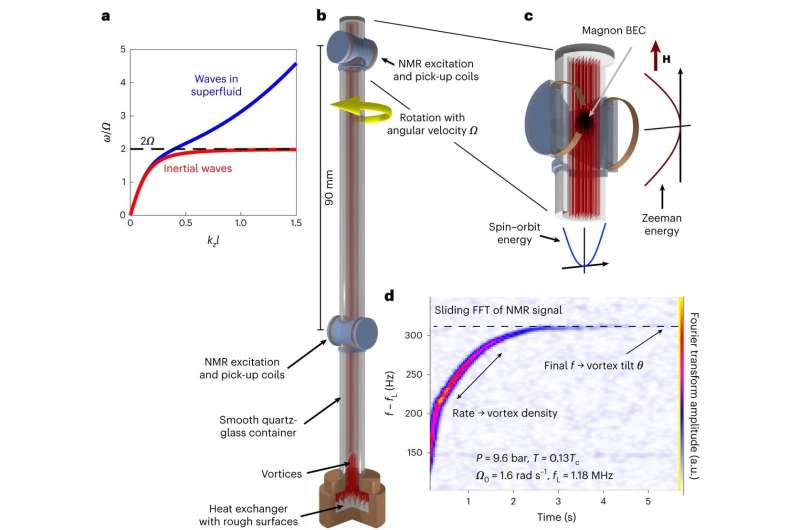
Most people only encounter turbulence as an unpleasant feature of air travel, but it's also a notoriously complex problem for physicists and engineers. The same forces that rattle planes are swirling in a glass of water and even in the whorl of subatomic particles. Because turbulence involves interactions across a range of distances and timescales, the process is too complicated to be solved through calculation or computational modeling—there's simply too much information involved.
Scientists have attempted to tackle the issue by studying the turbulence that occurs in superfluids, which is formed by tiny identical whirls called quantized vortices. A key question is how turbulence happens on the quantum scale and how is it linked to turbulence at larger scales.
Researchers at Aalto University have brought that goal closer with a new study of quantum wave turbulence. Their findings, published in Nature Physics, demonstrate a new understanding of how wave-like motion transfers energy from macroscopic to microscopic length scales, and their results confirm a theoretical prediction about how the energy is dissipated at small scales.
How energy disappears
The team of researchers, led by Senior Scientist Vladimir Eltsov, studied turbulence in the Helium-3 isotope in a unique, rotating ultra-low temperature refrigerator in the Low Temperature Laboratory at Aalto. They found that at microscopic scales so-called Kelvin waves act on individual vortices by continually pushing energy to smaller and smaller scales—ultimately leading to the scale at which dissipation of energy takes place.
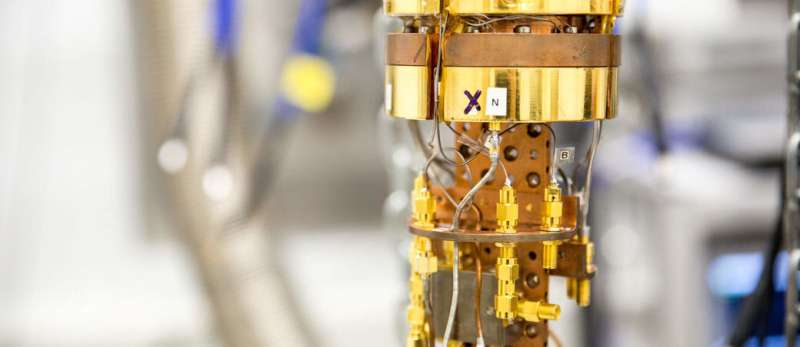
"The question of how energy disappears from quantized vortices at ultra-low temperatures has been crucial in the study of quantum turbulence. Our experimental set-up is the first time that the theoretical model of Kelvin waves transferring energy to the dissipative length scales has been demonstrated in the real world," says Jere Mäkinen, the lead author of the study and a Postdoctoral Researcher at Aalto.
Planes, trains and automobiles
In the future, an improved understanding of turbulence beginning on the quantum level could allow for improved engineering in domains where the flow and behavior of fluids and gases like water and air is a key question.
"Our research with the basic building blocks of turbulence might help point the way to a better understanding of interactions between different length scales in turbulence. Understanding that in classical fluids will help us do things like improve the aerodynamics of vehicles, predict the weather with better accuracy, or control water flow in pipes. There is a huge number of potential real-world uses for understanding macroscopic turbulence," Mäkinen says.
For now, Eltsov, Mäkinen, and others plan to go where the science takes them. Right now, their goal is to manipulate a single quantized vortex using nano-scale devices submerged in superfluids.
More information: J. T. Mäkinen et al, Rotating quantum wave turbulence, Nature Physics (2023). DOI: 10.1038/s41567-023-01966-z
Journal information: Nature Physics
Provided by Aalto University