This article has been reviewed according to Science X's editorial process and policies. Editors have highlighted the following attributes while ensuring the content's credibility:
fact-checked
peer-reviewed publication
trusted source
proofread
Scientists uncover key step in how diazotrophs 'fix' nitrogen
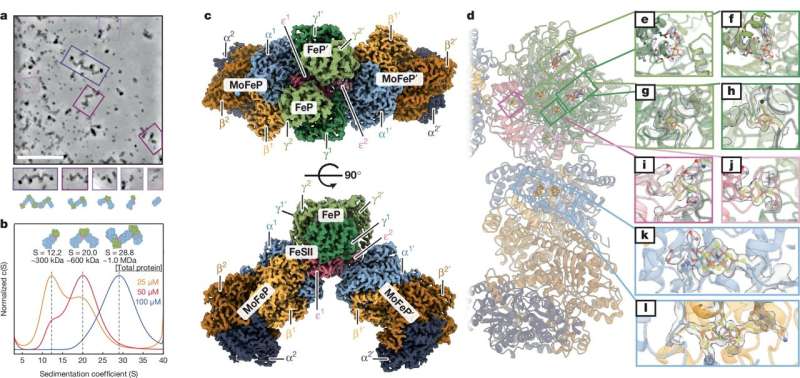
Nitrogen is an essential component in the production of amino acids and nucleic acids—both necessary for cell growth and function. Although the atmosphere is composed of nearly 80% nitrogen, this nitrogen is in the form of dinitrogen (N2), which cannot be processed by most organisms. Atmospheric nitrogen must first be converted, or "fixed," into a form that can be used by plants, often as ammonia.
There are only two ways of fixing nitrogen, one industrial and one biological. To better understand a key component of the biological process, University of California San Diego Professor of Chemistry and Biochemistry Akif Tezcan and Assistant Professor of Chemistry and Biochemistry Mark Herzik took a multi-pronged approach. Their work appears in Nature.
Thriving in a hostile environment
The industrial method is through the Haber-Bosch process, the advent of which in the early 20th century enabled the large-scale production of synthetic fertilizers, increased agricultural yields and helped the world population skyrocket. However, this process is energy-intensive, operating at very high temperatures and pressures. It also requires vast amounts of hydrogen, generated by burning fossil fuels, and is responsible for immense amounts of greenhouse gas emissions.
The biological route to fixing nitrogen is done by diazotrophs, nitrogen-fixing bacteria that contain an enzyme called nitrogenase. In contrast to the Haber-Bosch process, nitrogenase can be catalyzed at ambient pressures and temperatures and does not cause greenhouse gas emissions.
However, nitrogenase also requires large amounts of biochemical fuel, in the form of energy-producing adenosine triphosphate (ATP). Most diazotrophs make large amounts of ATP through cellular respiration by "burning" oxygen—even though nitrogenase is extremely sensitive to oxygen. Scientists have long wondered how a diazotroph, which needs to produce ATP by burning oxygen, also protects nitrogenase from being damaged by oxygen.
Diazotrophs have safety mechanisms in place; however, even with these protections in place, some oxygen penetration into the nitrogen-fixing cell is unavoidable. When this occurs, some diazotrophs employ a "method of last resort" to protect nitrogenase from destruction, called the conformational protection mechanism.
Here an iron-sulfur protein named FeSII senses increasing amounts of oxygen in the cell and binds to the nitrogenase enzyme complex, protecting it from damage and halting ammonia production. When the oxygen levels in the cell decrease, FeSII senses this again, disengages from the nitrogenase, and the ammonia production resumes.
Although scientists have long known of the FeSII protein, the mechanism by which it protected nitrogenase from oxygen damage was not understood. To answer these questions, the researchers employed several techniques, which together provided detailed insight into how this mechanism worked.
A robust toolkit helps answer a longstanding question
First author Sarah Narehood is a joint graduate student who splits her time between the Tezcan and Herzik labs, learning both the behavior of nitrogenase while also capitalizing on developments in cryogenic electron microscopy (cryoEM) technology.
"This work truly highlights the interdisciplinary advances necessary to elucidate the structural dynamics of these complex natural systems," stated Narehood.
The team used a cryoEM method called single-particle reconstruction that enabled them to take near atomic-level snapshots of a mixture of nitrogenase proteins in the presence of FeSII. Doing this, they were able to visualize the structures, clearly revealing that FeSII sandwiches between two other nitrogenase proteins, gluing them together into filaments. This sandwiching blocked oxygen from accessing the sensitive metal cofactors of the nitrogenase proteins, while also inducing dormancy.
While this explained how nitrogenase was protected from oxygen exposure, it didn't explain how FeSII was able to sense oxygen levels in the first place. To uncover this, the team used another technique called small-angle X-ray scattering (SAXS), using instrumentation located at the Stanford Synchrotron Radiation Lightsource.
The SAXS experiments showed that FeSII changes shape in the presence and absence of oxygen. In the presence of oxygen, FeSII's shape fit perfectly between the other proteins, sandwiching them together into filaments. When oxygen levels drop, FeSII relaxes its structure, leading to dissociation of the filaments and re-activation of nitrogenase.
The researchers also employed an analytical ultracentrifuge, essentially the "Formula 1" of centrifuges because of how rapidly it spins. In an analytical ultracentrifuge, protein particles sediment to the bottom at varying speeds—heavier particles sediment faster, while lighter ones fall more slowly. This method allowed the researchers to analyze the masses of the different proteins as further confirmation that when FeSII senses oxygen, it glues the other nitrogenase proteins into larger assemblies and filaments.
Now that they have uncovered the mechanism through which FeSII protects nitrogenase from oxygen harm, the team wants to catch it in action in living cells. To do this, they want to employ cryoEM torovidemography, which will allow for the 3D visualization of the whole diazotrophic cell while it is in the process of fixing nitrogen, as well as the in vivo mechanism of oxygen protection.
Despite the fact that nitrogenase is one of the most critical enzymes in the biosphere, the molecular details of how it functions are still largely unknown. Resolving the details of the conformational protection mechanism ps an important piece of the puzzle, with significant practical implications.
"If the nitrogenase machinery can be encoded into plant cells so they do not require artificial fertilizers, it could mean less greenhouse gas emissions without sacrificing agricultural yield," said Tezcan.
Something critically important for a growing population on a polluted planet.
More information: Akif Tezcan, Structural basis for the conformational protection of nitrogenase from O2 (N&V), Nature (2025). DOI: 10.1038/s41586-024-08311-1. www.nature.com/articles/s41586-024-08311-1
Journal information: Nature
Provided by University of California - San Diego